Trillions of bacteria, viruses, fungi, archaea and eukaryotic organisms live within the human gastrointestinal tract and make up what is collectively called the gut microbiome. These organisms represent over 50% of the cells found within the human body and weigh up to 2 kg in an average adult.1,2 The stomach and small intestine have relatively few organisms because of the acidic environment, the presence of bile and pancreatic juice, and the effects of peristalsis that limit stable colonisation. Thus, it is in the colon where the overwhelming majority (1012) of the microbiota live and interact with the human host. Our knowledge of the human gut microbiome in both health and disease has expanded rapidly in the past 10 years, driven by increased availability and reduced cost of gene sequencing.3 This has provided compelling evidence of the gut microbiome’s vital role in normal metabolism, nutrition, immune function, physiology and prevention of disease. In addition to direct actions on the gut mucosa and enteric nervous system, many chemical mediators produced by the gut microbiome enter the blood stream and communicate with distal organs such as the brain, heart and liver.4
Microbiome in health
Although there is no standard definition of a healthy gut microbiome, important characteristics are a microbiome with high levels of diversity, stability, resistance to stress-related change (antibiotics, infections, immunosuppression) and a high level of redundancy of metabolic pathways.5
The developing gut microbiome
Historically, the gut in utero was perceived as a sterile environment; however, emerging evidence suggests microbial contact begins prior to birth, from maternal microbiota.6 More extensive colonisation begins at birth and during delivery from protective vaginal microbes, such as Lactobacillus. Babies delivered via caesarian section and those not exclusively breastfed, however, may be colonised by skin and hospital-acquired bacteria, such as Staphylococcus and Acinetobacter, leading to a microbiome that is initially less diverse and less healthy.6,7 These infants seem more susceptible to developing asthma, allergic rhinitis, diabetes and coeliac disease.8 The developing gut microbiome in the first year is similar to that of the mother, but is soon influenced by a variety of factors, including diet, feeding habits and surrounding environment.9 The mature gut microbiome is established between the ages of one and three years and thereafter is relatively stable. By this time, it is primarily composed of anaerobic bacteria, with well over 50 phyla and 1000 species represented. Despite this diversity, the majority of microbes come from two bacterial phyla: Bacteroidetes and Firmicutes.10
Metabolic and signalling functions
The gut microbiome is responsible for a number of vital metabolic and signalling functions, many of which are not achievable by the human body alone. These include the synthesis of all B vitamins (B1–B12) and vitamin K,11 processing of food, digestion of otherwise indigestible complex polysaccharides (starches, cellulose and gums), synthesis of essential amino acids, biotransformation of bile to assist with glucose and cholesterol metabolism, and the production of short-chain fatty acid metabolites, such as butyrate and acetate, that act as an energy source for colonic bacteria and can exert anti‑inflammatory effects.12 Certain partially digestible foods can in turn enrich specific beneficial microbial populations, although a direct health benefit is yet to be established. Often known as prebiotics, examples of these foods include cereal grains, asparagus, leeks, artichokes, legumes, cabbage and kale.12 Gut microbiota can also communicate with the central nervous system through multiple pathways, including the vagus nerve, hypothalamic–pituitary–adrenal axis, the production of neurotransmitters or their precursors, including serotonin, tryptophan, gamma-aminobutyric acid, dopamine, l-dopa and noradrenaline, and via hormones, such as cortisol, ghrelin, leptin and glucagon-like peptide -1.13
Protective immune functions
Normal gut microbiota are our first internal line of defence against pathogens and toxins, protecting the body against disease in tandem with the host immune system. One of the key functions of a healthy microbiome is to prevent colonisation of pathogens through a barrier effect. This occurs through the production of antimicrobial compounds such as bacteriocin and the outcompeting of pathogens for nutrients and attachment sites through sheer force of numbers.3 Gut bacteria are also integral to the development of the mucosal innate immune system through direct interactions with intestinal epithelial cells. Early exposure to a variety of bacteria provides a kind of training for the immune system such that normal protective responses occur to commensals and appropriate inflammatory responses occur with exposure to pathogens. Conversely, reduced microbial diversity and exposure early in life can lead to an immune system that over-reacts to antigens, predisposing to autoimmune and allergic disease.14
Microbiome and disease
Many gastrointestinal diseases have been associated with alterations in the gut microbiome, as have metabolic disorders, liver disease, certain neurological and mood disorders, arthritis and immunological conditions.15 Dysbiosis, meaning an imbalance or maladaptive state of the microbiome, is found in almost all of these conditions, although whether this is cause or effect in most cases has not been established. We review those conditions with the most evidence and discuss research into modulation of gut bacteria as therapy.
Clostridium difficile infection
Clostridium difficile is a spore-forming, Gram-positive anaerobe, producing toxins A and B; the latter is responsible for colonic inflammation and the development of pseudomembranous colitis. The incidence and severity of C. difficile infection (CDI) in Australia and worldwide is on the rise, with over 12,000 cases reported in Australian hospitals in 2012, leading to an attributable mortality of 6–7%.16 C. difficile rarely causes disease in healthy adults; rather, it requires the impairment of normal resistance mechanisms and host flora, most commonly caused by broad-spectrum antibiotics, immunosuppression or the use of proton pump inhibitors.17
The traditional treatment for CDI has been with metronidazole or vancomycin, and removal of the offending trigger antibiotic; however, this is proving increasingly ineffective with recurrence rates up to 50% and increasing incidence of fulminant disease. Following a 2013 publication from the Academic Medical Center in Amsterdam,18 correcting underlying dysbiosis through faecal microbiota transplant (FMT) has been shown to be more effective and durable than antibiotic therapy for recurrent CDI. These results that have been replicated in Australia.19 The immediate impact of FMT in severe pseudomembranous colitis is illustrated in Figure 1.
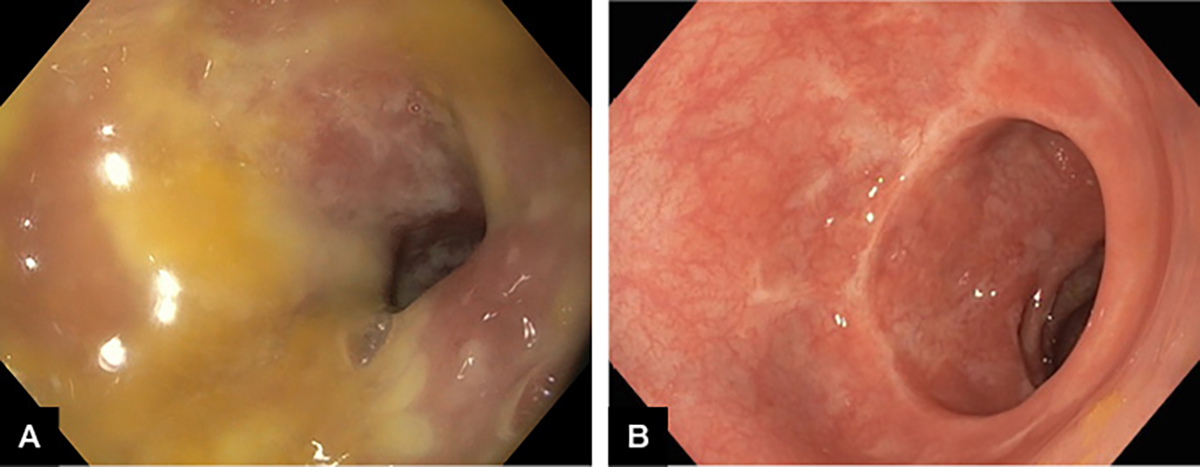
Figure 1. Severe Clostridium difficile infection in a patient aged 79 years.
A. Pseudomembranous colitis; B. Five days after faecal microbiota transplant
Inflammatory bowel disease
Ulcerative colitis and Crohn’s disease are chronic inflammatory conditions of unknown aetiology whose underlying pathogenesis appears to relate to multiple factors, including a genetic predisposition, environmental triggers, altered immune function and abnormal reactions to the gut microbiome. Dysbiosis is present in patients with inflammatory bowel disease (IBD), characterised by a higher ratio of pathogenic to commensal bacteria, reduced bacterial counts in areas of active inflammation, and areas of bacterial mucosal invasion, which is rarely, if ever, seen in healthy individuals.20 Modulation of gut bacteria with antibiotics such as ciprofloxacin and metronidazole is an established treatment to reduce postoperative recurrence of Crohn’s disease, while probiotics can be effective for IBD-related pouchitis following colectomy.21,22 A number of studies have looked at FMT as a treatment for IBD, but with conflicting results. A systematic review of small case series showed an overall efficacy of 36%, with Crohn’s patients more likely to respond than those with ulcerative colitis.23 An Australian randomised placebo-controlled study showed similar efficacy in ulcerative colitis (44% steroid-free remission), using an intensive protocol of FMT via colonoscopy and enemas over eight weeks.24 In two additional randomised controlled trials for ulcerative colitis, one showed no benefit over placebo,25 while in the other FMT achieved remission in 24%, compared with 5% with placebo.26
Irritable bowel syndrome
Irritable bowel syndrome (IBS) is common disorder characterised by abdominal discomfort and altered bowel habit in the absence of an underlying organic cause. The pathophysiology of IBS remains unclear, but is likely to be multifactorial, with altered motility and food processing, visceral hypersensitivity, genetic susceptibility and alterations in the gut microbiome being important.27 Individuals with diarrhoea-predominant IBS have dysbiosis characterised by lower Clostridium thermosuccinogenes phylotype expression,28 whereas those with IBS and constipation have an increase in bacteria that produce and use lactate, leading to higher sulphide and hydrogen production.29 Furthermore, IBS occurring after gastroenteritis is well recognised (post-infectious IBS) and affected individuals have alterations in Bacteroidetes and Clostridia, compared with healthy controls.30 Altering gut bacteria with antibiotics can help, and rifaximin, the most well studied, has been shown to have a modest symptomatic benefit in diarrhoea-predominant IBS. This has led to its approval in the US, but not Australia, for this indication.31 A systematic review and a meta-analysis found that probiotics have modest beneficial effects on global IBS abdominal pain, bloating and flatulence scores, with combination regimens more effective.32 Because of heterogeneity of studies, specific beneficial strains or dosage regimens could not be isolated. A low FODMAP diet, aimed at reducing fermentable oligosaccharides, disaccharides, monosaccharides and polyols, has been shown to be effective for IBS but may itself restrict faecal bacterial abundance, including beneficial bacteria.33
Metabolic disease
The worldwide epidemic of obesity and associated metabolic disease has primarily been attributed to diet and lack of exercise; however, it is now clear that the gut microbiome is also implicated as both friend and foe. Gut microbiota are required for diet-induced weight gain (Figure 2A), with germ-free mice gaining minimal weight despite a high fat/sugar diet, whereas identical mice containing gut bacteria become obese.34 Dysbiosis characterised by reduction in the abundance of Bacteroidetes and an increase in Firmicutes, reversing their normal ratio, has been implicated in murine and human obesity;35 however, two recent meta-analyses of pooled human studies failed to identify these changes in bacterial phyla, with only minor reductions in microbial diversity and richness seen.36,37 Nevertheless, obese and lean metabolic phenotypes in mice can be transmitted via FMT (Figure 2B),38,39 a process more dependent on microbiota than genetics (Figure 2C), as FMT from identical twins discordant for obesity still led to transmission of the lean or obese phenotype in mice.40 Co-housing the two groups, where mice ate each other’s faeces, led to a lean phenotype, hypothesised to be due to re-invasion of specific Bacteroidetes into obese mice.40 In a recent study in patients with metabolic syndrome, FMT from a lean donor improved insulin sensitivity in just six weeks, an effect in part driven by increased butyrate-producing gut bacteria.41
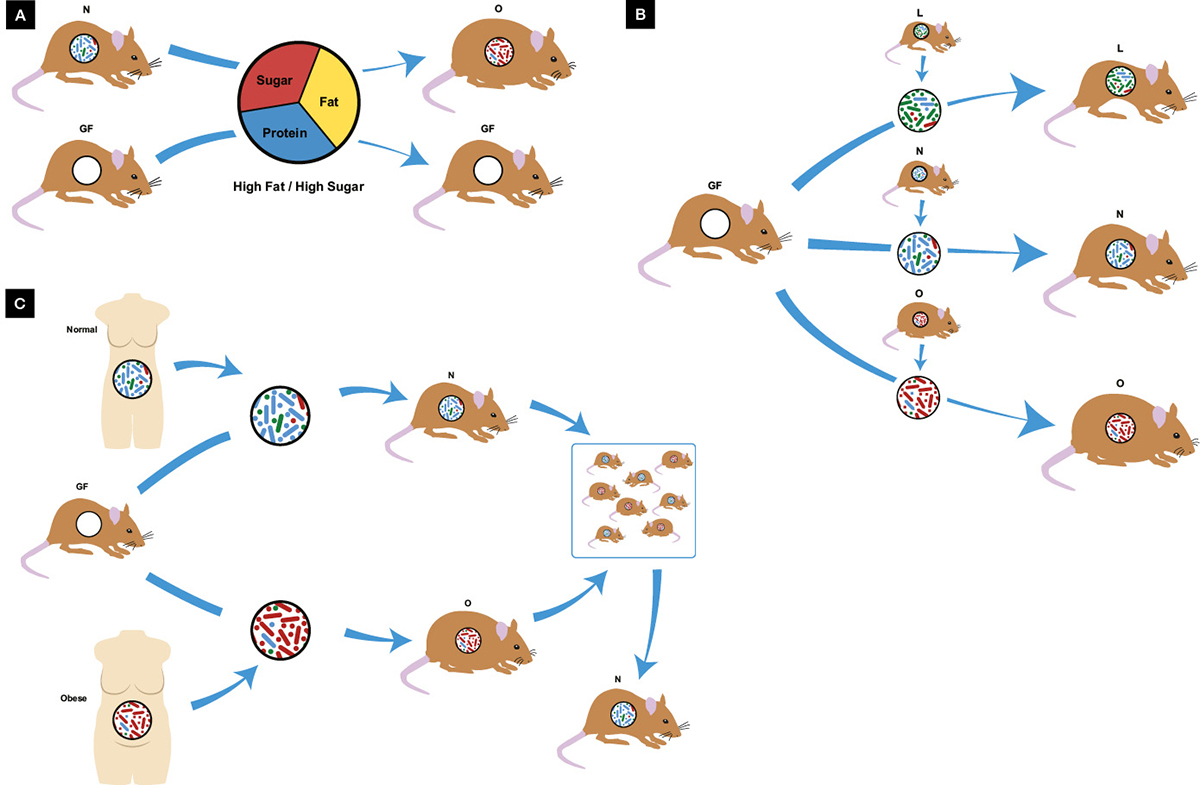
Figure 2. Obesity and the microbiome
A. Germ-free mice are resistant to diet-induced obesity; B. Metabolic phenotype is transmissible via microbiota transplant with lean donors producing lean mice and obese donors producing obese mice; C. Microbiota transplant from identical twins discordant for obesity also transmit metabolic phenotype to germ-free mice, however when co-housed the lean phenotype predominates; GF, germ-free; L, lean N, normal; O, obese
Non-alcoholic fatty liver disease
In addition to the microbiota changes of obesity, alterations in gut bacteria in non-alcoholic fatty liver disease (NAFLD) and non-alcoholic steato-hepatitis (NASH) are associated with intestinal bacterial translocation, leading to increased delivery of lipopolysaccharides, free fatty acids and other toxins directly to the liver from the portal vein.42 Furthermore, patients with NASH have a greater abundance of the Escherichia family of bacteria, a group that ferments carbohydrates and produces endogenous ethanol.43 This leads to increased alcohol levels peripherally, a possible co-factor in the pathogenesis of fatty liver disease. A number of studies are underway to assess the role of probiotics, FMT or other gut-modulating procedures in patients with NAFLD.
Altering the gut-microbiome
Faecal microbiota transplant
FMT was first used in modern medicine in 1958, when four patients with pseudomembranous colitis were cured by faecal enema.44 Evidence exists for the role of FMT in recurrent CDI and there is emerging evidence for its role in IBD and metabolic disease.15 The process behind FMT is relatively simple. Donors are screened for all known bloodborne and stoolborne pathogens and must be healthy, lean and free of any diseases potentially transmissible via gut bacteria. Donated stool is blended with sterile saline/glycerol and can be given fresh or frozen. Stool frozen at –80°C is viable and effective for at least six to nine months.19 Donor stool can be delivered via naso-jejunal tube or into the caecum at colonoscopy. Overall, the procedure is safe; common adverse events include bloating, diarrhoea and low-grade fever, which tend to settle within 48 hours.15 Serious events related to the endoscopic procedure, such as bowel perforation and aspiration, are rare. The long-term effects of FMT are not known and could plausibly include induction of chronic diseases related to alterations in gut bacteria or transmission of currently unrecognised infectious agents.15 Researchers from the OpenBiome stool bank in the US, among others, have developed a faecal capsule, which may make this gut-changing therapy more accessible in the future.
Antibiotics
Manipulating gut bacteria with antibiotics such as ciprofloxacin and metronidazole has been used to treat intestinal disorders such as Crohn’s disease for some time; however, their ability to reduce pathogenic Gram-negative bacteria, parasites and anaerobes comes at the cost of reducing bacterial diversity and resistance, and CDI.12 Recently, the minimally absorbed broad-spectrum antibiotic rifaximin has gained favour for its efficacy in hepatic encephalopathy and IBS, and low potential for developing bacterial resistance.12
Probiotics
Probiotics are defined by the World Health Organization as ’live bacteria or yeasts that, when administered in adequate amounts, confer a health benefit on the consumer’. However, evidence for their efficacy is mixed. The most well-studied bacterial probiotics are those of the Lactobacillus, Bifidobacterium and Lactococcus species, while the most commonly used yeast is Saccharomyces boulardii.12 Probiotics colonise the gut temporarily and may exert beneficial effects through enhancement of the natural intestinal barrier (both physical and mucous layer), stimulation of IgA secretion, downregulation of inflammatory cytokine production and direct antagonism of pathogens.45 On the basis of meta‑analyses of randomised controlled trials, probiotics are modestly effective for reducing global symptoms in IBS (number needed to treat [NNT] = 8),32 and in reducing antibiotic-associated diarrhoea in children aged one month to 18 years (NNT= 10)46 and adults up to age 64 (relative risk = 0.47), but not in the elderly.47 The duration of probiotic use (5–21 days) did not alter the benefit and, to varying degrees, results were strain-specific; the biggest benefit was conferred from Lactobacillus and S. boulardii.47 For other diseases, results may be preparation‑specific, with only the expensive combination probiotic VSL#3 (eight species of Lactobacillus, Bifidobacterium and Streptococcus; $130) being effective for prevention and treatment of pouchitis in IBD following colectomy,22 and Lactobacillus casei and a mixed research preparation of L. acidophilus, L. delbruecki var bulgaris, Streptococcus thermphilus and B. bifidu for five days reducing symptom duration and severity in children with acute infectious diarrhoea.48 Evidence that probiotics assist in extra-intestinal disease, or to help maintain general health, remains controversial. Most commercial probiotics consist of a blend of strains with efficacy generally attributed to specific strains and their quantity. However, a recent study revealed that only 58% are correctly labelled.49 Furthermore, there are issues with maintaining the viability of probiotics during storage, and their ability to withstand acid and bile to reach the colon where colonisation can occur.50 While probiotics appear to be safe in the majority of individuals, case reports have raised the possibility of opportunistic infection due to interference with commensal microflora, particularly in critically ill adults and neonates, where bacteraemia and fungaemia have been occasionally reported after administration of Lactobacillus rhamnosus and S. boulardii.51 Large randomised trials have not confirmed an increased risk; however, a risk-benefit assessment is recommended when probiotics are used.
Conclusion
The gut microbiome is a complex and essential part of our bodies that provides vital support for normal metabolic function and protection against illness. Dysbiosis is associated with multiple disease states and is a target for therapy and future research.
Acknowledgements
The authors wish to thank Mr Andrew Barnes for his assistance with the artwork for Figure 2.
Authors
Mayenaaz Sidhu MBBS, Advanced Trainee Gastroenterology, Westmead Hospital, Sydney, NSW
David van der Poorten BSc (Med) MBBS (Hons) FRACP PhD, Staff Specialist Gastroenterologist Westmead Hospital, Sydney, NSW and Clinical Associate Professor, University of Sydney, Sydney, NSW. david.vanderpoorten@sydney.edu.au
Competing interests: None
Provenance and peer review: Commissioned, externally peer reviewed.