Lung cancer is the leading cause of cancer-related death in Australia, placing substantial burden on medical and economic resources.1 Non–small cell lung cancer (NSCLC) is the most common subtype of lung cancer, and treatment options depend on the American Joint Committee on Cancer (AJCC) stage at presentation.2 Management can be complex because many patients present with metastatic or inoperable locoregionally advanced disease, and have medical comorbidities that limit the tolerability of treatment. Radiation therapy has an integral role across all stages of the disease (Table 1).
Table 1. Overview of standard and emerging treatment options for NSCLC according to American Joint Committee on Cancer Stage
Stage
|
Standard
|
Emerging
|
Benefit
|
Stage I–IIa
(Primary <7cm)
|
- Surgical resection
- Conventional fractionated radiation therapy (up to 33 daily treatments)
|
- SABR (1–5 daily treatments)
|
- Reduced morbidity
- Convenience
- Improved local control if inoperable
- Expands curative options
|
Stage IIb–III
(Primary >7 cm or extensive local invasion or nodal involvement)
|
|
- IMRT
- 4DCT planning
- Respiratory gating/4DRT
|
- Reduced morbidity
- Theoretically improved therapeutic window
|
Stage IV
(Brain metastases)
|
|
- Stereotactic radiosurgery or radiotherapy
- Hippocampal-avoidance WBRT
|
- Non-invasive treatment to improve local control, reduced morbidity
- Maximises distant intracranial control with less toxicity
|
Stage IV
(Limited metastatic disease [oligometastases])
|
- Chemotherapy
- Targeted therapy
- Simple radiation therapy
|
- SABR for oligometastases
- Sequencing with immunotherapy
|
- Improves local control of symptomatic metastases
- Theoretically prolongs effectiveness of systemic therapy, survival
|
3DCRT, three-dimensional conformal radiotherapy; 4DCT, four-dimensional computed tomography; IMRT, intensity-modulated radiotherapy;
SABR, stereotactic (ablative) body radiotherapy; WBRT, whole brain radiotherapy
|
Advances in radiotherapy technology have the potential to improve outcomes for patients by enabling dose escalation to tumours, greater sparing of normal tissues and improved targeting of tumours that move with respiration. In this article, we review these technological advancements as they apply to NSCLC and provide general practitioners (GPs) with an update that may be of benefit to their patients.
Radiotherapy advances
Stereotactic (ablative) body radiotherapy for stage I–IIA disease
In Australia, lobectomy remains the standard of care for medically suitable patients with technically operable early-stage lung disease. Expected local control rates in these patients are 80–90%.3 For patients who are not suitable for surgery, fractionated radiation therapy delivered over six weeks is a less effective treatment with expected local control rates of 30–70%.4,5
Stereotactic (ablative) body radiotherapy (SABR) is a specialised technique that builds on radiosurgical processes initially developed to treat intracranial targets. It enables the precise delivery of high radiation therapy doses per fraction while limiting dose to surrounding normal tissues. SABR has the potential benefits of local control rates comparable to surgery, low morbidity, and convenience for patients who refuse or are unfit for surgery.5,6 Treatment is painless and usually delivered over one to five sessions (fractions), each lasting less than one hour. Patients can resume normal activities shortly afterwards.5
SABR has increased the number of patients who can be treated with curative intent.7 Rates of severe radiation pneumonitis are low at 3%, even in patients with compromised lung function,4–6 compared with 13–37% in patients receiving conventional radiation therapy.8 Figure 1 illustrates the differences in dosimetry achieved using the two radiation therapy techniques.
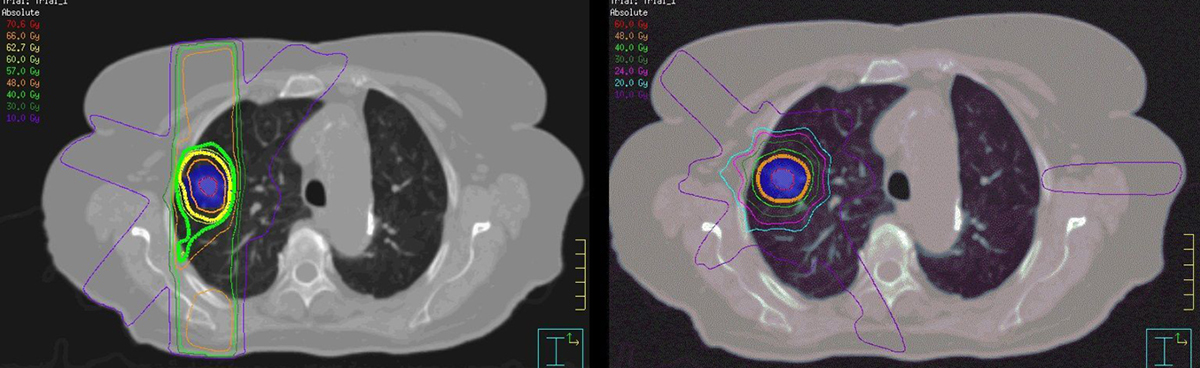
Figure 1. Conventional radiotherapy versus SABR
Axial view of dosimetry with conventional fractionated 3DCRT (left) and SABR (right) for the same stage INSCLC patient. The coloured lines represent radiation dose distribution, with the thick yellow line representing the prescribed dose. SABR delivers a biologically more effective dose to the tumour (blue shading) and the dose to surrounding tissues is less.3DCRT, three-dimensional conformal radiotherapy; NSCLC, non-small cell lung cancer; SABR, stereotactic (ablative) body radiotherapy.
The only two randomised controlled trials (RCTs) comparing SABR with surgery in medically operable patients failed to accrue and closed prematurely. A pooled analysis of their data has suggested statistically superior survival rates for those treated with SABR.9 It is postulated that this observation is because of reduced risk of treatment-related death, but this conclusion remains controversial and further studies are warranted.
SABR for oligometastatic disease
For most patients with metastatic NSCLC, the prognosis is poor. However, some patients with limited disease (up to five metastases) may have a more indolent tumour biology and a more favourable prognosis. These patients are said to have ‘oligometastatic disease’ and may benefit from local ablative therapy.2 As a non-invasive, outpatient alternative to surgery, SABR can be used in this setting to achieve local control rates of >80% with low toxicity.10
Non-randomised data suggest that the combination of SABR and erlotinib in patients with oligometastatic disease improves progression-free and overall survival, compared with historical controls (14 and 20 months versus two and six months, respectively),11 but high-quality data are lacking. Potentially beneficial synergistic interactions between SABR and systemic agents is an area of active research.12 SABR could be used to ablate self-selected tumour foci that develop resistance to targeted therapy, or precipitate release of antigens that immunotherapy can exploit to stimulate an augmented response.
Intensity modulated radiation therapy for stages II–III disease
The standard treatment for inoperable locoregionally advanced disease is chemoradiotherapy.13 Radiotherapy is typically delivered in 30–33 daily fractions at five per week to a total dose of 60–66 Gy.3 Distant metastatic failure is the greatest risk for these patients, but up to 46% may have persistent or recurrent locoregional disease as well.14 SABR is not possible in this setting because the volume is large and mediastinal structures are included in the treatment volume.
Intensity-modulated radiation therapy (IMRT) is an established radiation therapy technique routinely use in other cancer subsites (eg head and neck cancer) and delivers improved radiation dose shaping, compared with standard three-dimensional conformal radiotherapy (3DCRT). IMRT relies on multiple, computer-controlled, independently driven tungsten leaves to variably block parts of the beam and improve the conformity of the high and intermediate doses to the target. This might improve outcomes through reduced toxicity in normal tissues and/or dose escalation to the tumour.15 In Australia, 3DCRT remains the standard of care in most hospital departments, but IMRT may be considered on an individualised basis to treat large targets or those close to critical structures. Figure 2 illustrates an IMRT treatment plan in a patient with Stage III NSCLC.
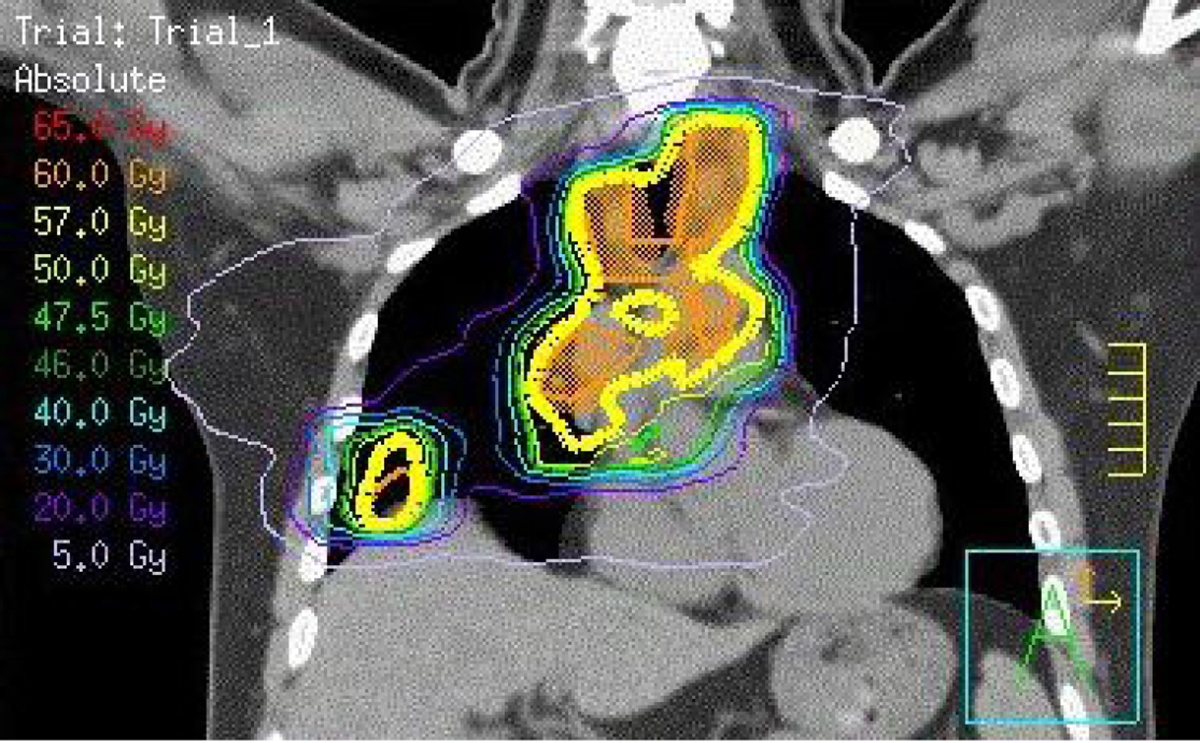
Figure 2. Coronal view of IMRT dosimetry
Coronal view of intensity-modulated radiotherapy dosimetry demonstrating conformity of the prescribed dose (yellow line) to the target volume (orange shading) while sparing lower dose to lung and heart (blue and purple lines).
IMRT, intensity-modulated radiation therapy
Motion management strategies
Four-dimensional computed tomography and radiation therapy
Tumours in the lungs move with respiration during treatment, particularly when they are located close to the diaphragm. To ensure that the tumour receives the intended dose, the convention is to add a margin of normal tissue around the target to account for this uncertainty.
Four-dimensional computed tomography (4DCT) is a specialised scanning technique used during the radiation therapy planning stage to characterise and quantify individual tumour movement during respiration. This technique is essential when using sophisticated techniques in the chest such as SABR or 3DCRT/IMRT that deliver high doses with steep dose gradients. By accurately defining the tumour volume in every phase of respiration, 4DCT reduces the risk of geographical miss and can spare unnecessary irradiation of normal lung through an individualised approach.16
The four-dimensional concept can also be applied to radiation therapy. Through the use of fiducial markers on the patient or within the tumour, treatment can be delivered in a way that continuously responds to respiratory motion in real time. This is achieved using various techniques, including a robotic arm–mounted linear accelerator (eg Cyberknife), a robotic couch or dynamic tungsten leaves that variably block parts of the field while the beam is on.17,18 These technologies are not currently available in the majority of Australian radiotherapy departments.
Respiratory gating
Radiation therapy delivery can also be restricted to specific phases of the respiratory cycle with the use of gating technology, effectively treating only when the target moves into the crosshairs. Breathing training may be required to help patients regulate respiration.18 Respiratory gating further reduces the amount of normal lung tissue irradiated during treatment, but markedly prolongs the time required to deliver it.19 In practice, the additional gains in potential normal lung sparing may not be clinically or financially justifiable when alternative four-dimensional approaches are in use already. In addition, not all tumours have a high degree of motion, particularly those in the upper and medial regions of the thorax.18
Stereotactic radiosurgery for brain metastases
Up to 20% of patients with stage III NSCLC will develop brain metastases,20 and this may increase with the growing use of imaging in asymptomatic patients and the emergence of increasingly effective systemic therapies. The prognosis for brain metastases varies according to age, performance status, number of brain metastases and extent of extracranial disease.21,22
A standard treatment for patients with multiple metastases is whole brain radiotherapy (WBRT) with a median survival of four to six months.21,22 In select patients with better prognoses, local therapy with surgery or stereotactic radiosurgery (SRS) improves outcomes, compared with WBRT alone.23,24 SRS refers to the precise delivery of a single, high radiation therapy dose to the tumour to maximise local control and minimise dose to surrounding normal brain (Figure 3).
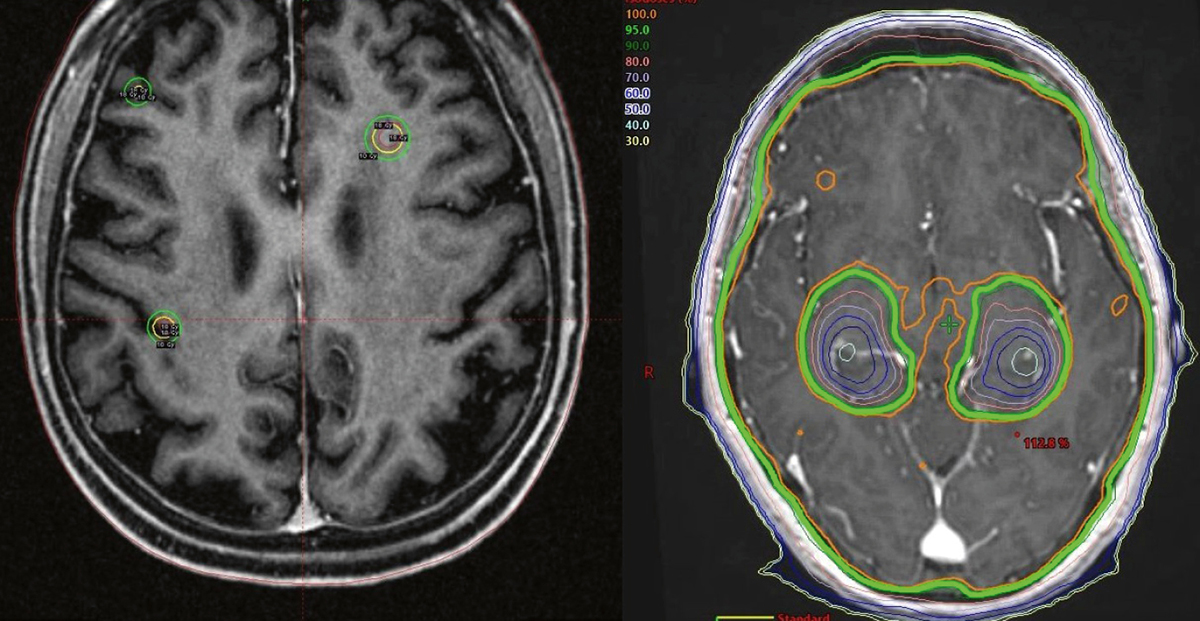
Figure 3. Treatment of brain metastases: SRS and HA-WBRT
Left – axial view of Gamma Knife SRS plan to three non-small cell lung cancer brain metastases.
The metastases receive a high dose (yellow line) that rapidly drops away such that the rest
of the brain receives a very low dose.
Right – HA-WBRT dosimetry demonstrating homogenous coverage of the brain with the prescribed dose (green line) except in the medial temporal lobes, with sparing of bilateral hippocampi. Significant dose dropoff around both hippocampi (coloured lines) is demonstrated.
Outcomes following surgery or SRS are considered comparable for lesions <2–3 cm in diameter, but they have never been compared successfully in an RCT. Surgery may be preferred for larger symptomatic lesions or when histology is required. SRS is painless and permits treatment of multiple targets in a single outpatient treatment. Patients are immobilised using an invasive headframe or thermoplastic shell.
The addition of WBRT after local therapy improves radiological measures of intracranial control, but the clinical benefit is unclear and it may be associated with adverse quality-of-life and neurocognitive effects.25–27 Withholding WBRT after SRS with magnetic resonance imaging (MRI) surveillance is now a standard treatment approach.2
Following surgery, local control is approximately 40% at two years.28 SRS or stereotactic radiotherapy (SRT) to the surgical cavity may be considered as an alternative to WBRT to improve local control.2 Distinct from SRS, SRT is delivered over three to five fractions to exploit the principle of fractionation and improve the therapeutic ratio (similar to SABR in the body).
Hippocampal-avoidance WBRT
Although WBRT may be associated with toxicity, uncontrolled intracranial disease poses the greatest risk to patients with brain metastases. Thus, novel ways to deliver WBRT have been explored. Preclinical data suggest that the deleterious effects of cranial radiation therapy on neurocognitive function are at least in part related to effects on neural stem cells located in the subventricular zones lining the lateral ventricle and hippocampal dentate gyrus.29 Using IMRT, the dose to these structures can be reduced but maintained elsewhere to deliver so-called hippocampal avoidance WBRT (HA-WBRT). An example of this is given in Figure 3.
In a non-randomised prospective study of 42 patients with multiple brain metastases at least 5 mm from either hippocampus, the risk of memory decline at four months after HA-WBRT was 7%, compared with a historical control of 30% after standard WBRT and quality-of-life measures remained stable.30 Whether the additional training, costs and resources required to deliver HA-WBRT can be justified in all patients is a matter of ongoing debate.
Conclusion
NSCLC is a challenging disease to treat and has historically poor outcomes. Radiation therapy has an important role across all stages of the disease. Technological advances have expanded treatment options for appropriately selected patients, to improve the quality and quantity of survival. Equipped with this knowledge, GPs can more effectively inform, support and refer patients as part of the multidisciplinary team needed to treat NSCLC.
Authors
Michael Huo BCom, MBBS, Radiation Oncology Registrar, Department of Radiation Oncology, Princess Alexandra Hospital, Woolloongabba, Qld; School of Medicine, University of Queensland, Brisbane, Qld. michaelhuo@live.com.au
Peter Gorayski BSc (Hons), BMBS, FRACGP, FRANZCR, Radiation Oncologist, Radiation Oncology Centres, Mater Private Hospital Springfield, Springfield Central, Qld; School of Medicine, University of Queensland, Brisbane, Qld
Mark B Pinkham BMBCh, MA (Hons), FRANZCR, Radiation Oncologist, Department of Radiation Oncology, Princess Alexandra Hospital, Woolloongabba, Qld; School of Medicine, University of Queensland, Brisbane, Qld
Margot Lehman MBBS (Hons), GDPH, FRANZCR, Senior Radiation Oncologist and Director, Department of Radiation Oncology, Princess Alexandra Hospital, Woolloongabba, Qld; School of Medicine, University of Queensland, Brisbane, Qld
Competing interests: None.
Provenance and peer review: Not commissioned, externally peer reviewed.
Acknowledgements
We would like to acknowledge Brock Lamprecht and Angela Coles, Radiation Therapists, Princess Alexandra Hospital, Woolloongabba, Queensland, Australia for their assistance with Figures 1 and 2.